Tracking the environmental dimensions of armed conflicts from space.
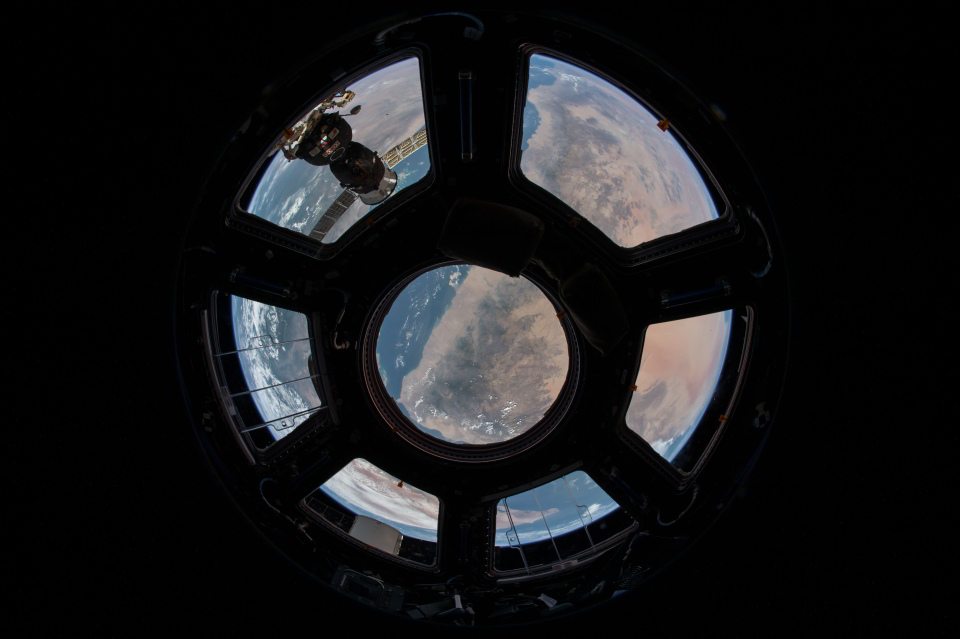
Regions of active conflict are typically data poor, access for environmental measurements is limited and many contemporary conflicts show no sign of conclusion. Earth observation (EO) via satellite remote sensing can fill these data gaps and provide a wealth of information ranging from short-term environmental risks to long-term changes. All this information can help target locations for humanitarian response, remediation or in-situ data collection via citizen science or post-conflict environmental assessments. Observations can help form the basis of environmental peacebuilding or could even help hold those responsible for environmental crimes to account.
What can we measure from space and how?
Most sensors are passive, meaning that they rely on the Sun’s energy to illuminate the Earth and the atmosphere and then measure the return signal. This energy could be directly reflected at visible wavelengths, or absorbed and re-emitted at infrared, ultraviolet and microwave wavelengths. Different surface objects and atmospheric compounds re-emit this energy at different wavelengths, so each one has its own spectral ‘fingerprint’. Sensors aim to capture this information by measuring multiple or very specific wavelengths.
Landsat, Sentinel-2 and MODIS are among the key passive sensors providing myriad imagery and data on, amongst others, land and sea surface temperature, surface water, vegetation health, fire activity, deforestation, desertification and pollution. Of the atmospheric constituents of interest, only those with the strongest signals can be retrieved; key sensors include TROPOMI (carbon monoxide, formaldehyde, methane, nitrogen dioxide, ozone, sulphur dioxide), GOSAT (carbon dioxide, halocarbons, methane, nitrous oxide) and MODIS (aerosol type and optical depth).
With the exception of anthropogenic night-time lights, passive sensors cannot provide data when it is dark or cloudy. At these times, active sensors – radar and lidar – are invaluable as they do not rely on the Sun, instead transmitting their own narrow band of energy towards the Earth. Radar sensors can measure surface topography via altimetry (height above sea level; eg Sentinel-3); precipitation structure via Ka-band radar (eg GPM); and trees, leaves and surface elevation via synthetic aperture radar (SAR) at L-band (PALSAR), C-band (Sentinel-1) and X-band (TanDEM-X) respectively. Interferometric-SAR (InSAR) is the practice of combining SAR data to monitor ground movements. Lidar sensors help us understand canopy structure (GEDI) and the vertical distribution of atmospheric winds (AEOLUS) and aerosols (eg CATS).
Most EO satellites fly in a low (700 km) Sun-synchronous orbit, ie they observe the same scene at the same angle and illumination. The size of the scene, called the ‘swath’, ranges from metres to hundreds of kilometres and determines the time between revisits to the same surface location. To complete a full Earth survey may take days to months with smaller swaths and hence, unless fortunate, they are of limited use for incident monitoring. The compromise for sensors with a larger swath is a reduction in spatial resolution. At the extreme, sensors in geostationary orbit, ie moving in sync with the Earth’s rotation to look at one area, can provide rapid data (eg every 15 minutes with SEVIRI) but at low spatial resolutions as they have to fly at high altitude (36,000 km).
Using EO data in conflict settings
As the environmental consequences of conflict can be wide ranging and pervasive, the majority of EO satellites are useful. The difficulty is in knowing which are available, can be easily accessed, quickly processed and trusted to draw robust conclusions from. EO sensors can also help us understand environmental changes that may contribute to conflicts – be they natural or anthropogenic – and that may be useful for environmental peacebuilding post conflict or for improving predictions of potential conflict hotspots.
Most EO data are open source and freely available. It is easy to forget how incredible this democratisation of data is, and without it, the world and our understanding of it would be much poorer. Increasingly, commercial satellite providers provide free high-resolution imagery for disaster monitoring. Derived products integrate multiple satellite and/or model data streams to provide more detailed and systemic information.
The best understanding arises when these satellite data are integrated with open-source intelligence (OSINT), a catch-all term that includes humanitarian data, social media, traditional media, georeferencing of event images and videos, and forensic architecture. Together these datasets let us identify the link between conflict and the environment, rather than just environmental change in a conflict zone. Sometimes this is clear and obvious but often it requires carful untangling, as is now explored through examples of recent work here at the Conflict and Environment Observatory.
Fast response incident monitoring
A significant part of our work is searching social and traditional media to find reports and locations of potential incidents. The aim is to quickly assess the veracity of these claims – environmental disinformation is rising in this fake news era – and characterise the scale and risk of the incident.
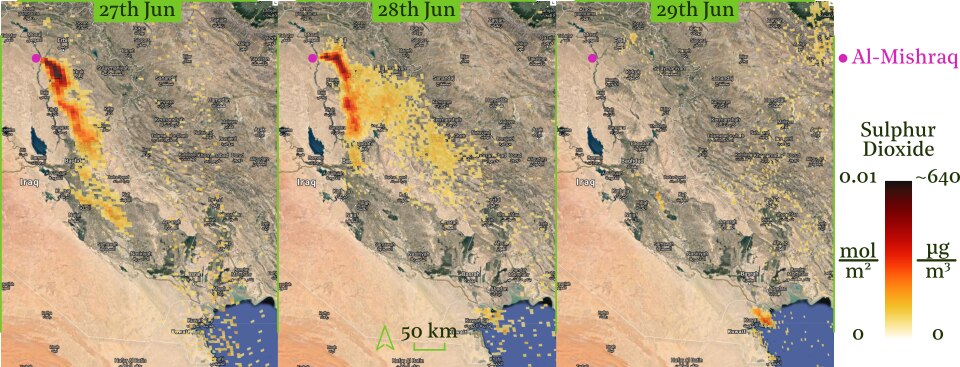
For example, on the evening of 26 June 2019, reports emerged that belligerents had set alight stockpiles of purified sulphur at the Mishraq Sulphur State Company in northern Iraq, resulting in the release of a significant near-surface plume of sulphur dioxide. Concern was significant, given that two previous fires, burning for 18 days in 2003 and seven days in 2016, had resulted in deaths and hundreds of hospital admissions in nearby Mosul, Erbil and Kirkuk. The latter looked vulnerable, based on quick online dispersion forecasts using the HYSPLIT model. Furthermore, if the entire sulphur stockpile were to burn and be released at high altitude, we estimate the amount of sulphur dioxide released would be on the scale of the 1991 Mount Pinatubo volcanic eruption – with a similar reduction in the quality and quantity of sunlight, affecting the global surface temperature alongside agricultural and economic productivity. The risk was heightened given the record number of crop fires in the region, linked to various belligerents but mainly Islamic State, and limited firefighting capacity.
Active fire data allowed confirmation of fires in the facility’s immediate vicinity on the same day. The next day, the Aura sensor provided the first data verifying a significant sulphur dioxide release, via the NASA Worldview platform, just hours after its overpass at 13:30 local time. The higher-resolution TROPOMI sensor allowed a more detailed insight into the plume structure when the data were released approximately a day later and processed via Google Earth Engine (a cloud computing service free to researchers). Fortunately, especially as concentrations were akin to the previous burns, the plume centreline missed urban areas and was fully extinguished within three days (see Figure 1).
Given the cloudless skies, the scale of the incident and lack of other local sources, this incident was relatively easy to track. Oftentimes data are less accessible and the story more difficult to interpret. A year after the incident there are additional and more robust data available to use in an ongoing project to forensically assess the incident: the how/why/where/who of the fire, the sulphur emitted and the agricultural and hydrological impacts of this plume, in addition to those from previous fires at the site. Given the regional security situation, new and more significant fires are possible, so we remain attentive.
Monitoring long-term changes
Environmental issues can drive and sustain conflicts, be they small or large, direct or indirect. Alternatively, or additionally, cumulative environmental degradation during a conflict can lead to conditions that prevent a sustainable peace. In all cases, it is imperative to properly characterise environmental changes.
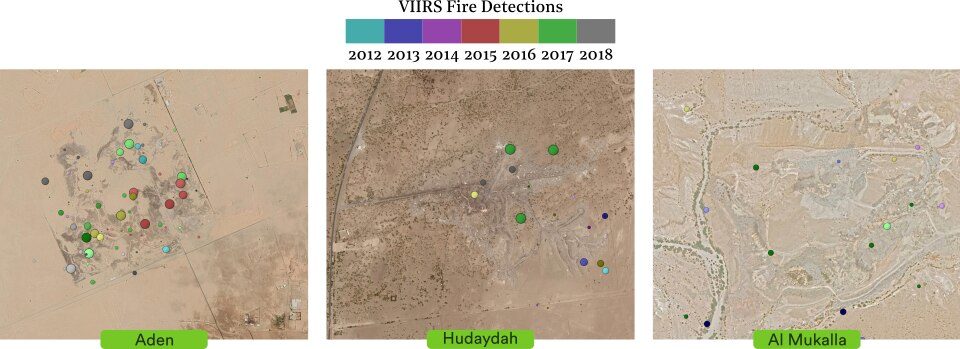
The consequences of conflict in Yemen have been severe for the environment: falling groundwater levels, farm abandonment, direct attacks on infrastructure and workers, collapse of wastewater management, deforestation and the introduction of alien species to the Socotra archipelago. Figure 2 shows informal waste dumps, identified from satellite using fire hotspot data from VIIRS. Landfills are a source of highly flammable methane, the presence of which can result in spontaneous accidental fires; methane can also sustain fires that are intentionally started to reduce waste volumes. Informal landfills have proliferated during the conflict as governance has collapsed, yet how waste is managed has implications for the protection of civilian health, their livelihoods and the environment.
EO is essential for monitoring and, when integrated with other datasets, can yield a fuller understanding of stories across active conflicts. While the higher-specification sensors launched in the past few years are powerful, it is often the sensors that have been in place for two decades or more that are the most useful, providing the longer time series essential for these analyses. For example, Figure 3 shows some of the long-term datasets we are currently using in our ongoing research into South Sudan at the Conflict and Environment Observatory. Here we focus on the Sudd wetlands (see Figure 3A), which provide the only palatable grasslands in the dry season for the cattle of pastoralist tribes. As the area of the wetlands changes, so too does the available grazing area, which can lead to tensions between tribes and with agriculturalists. This is in addition to the state violence and country-wide conflicts.
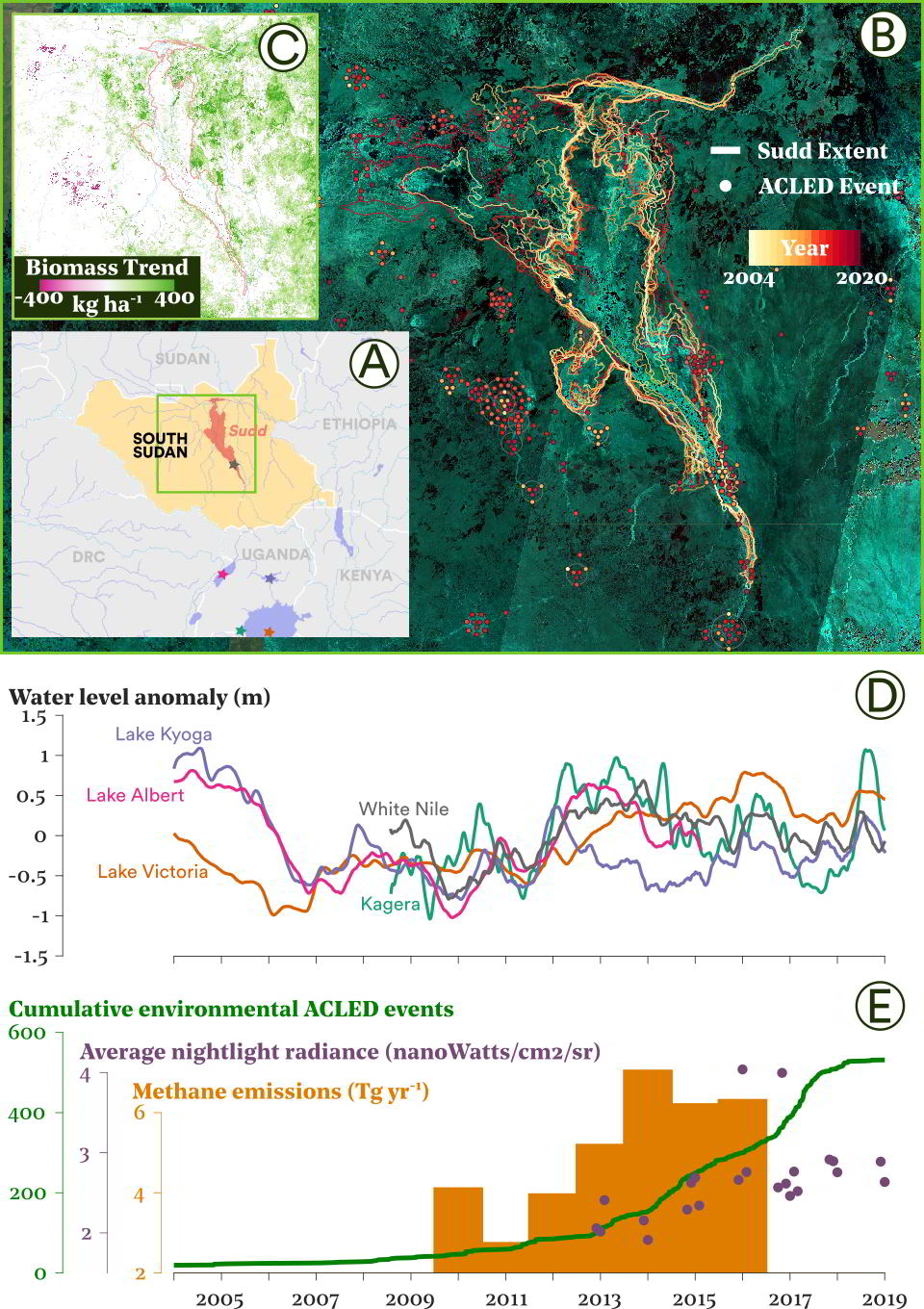
To untangle these complex interactions, the first step is to understand environmental changes, as these are little studied:
- Determination of the wetland extent using diurnal land surface temperature gradients (via MODIS emissivity retrievals, see Figure 3B);
- Understanding the hydrology upstream using precipitation data (via GPM precipitation radar) and lake/river levels (via JASON satellite altimetry, see Figure 3D), coupled to watersheds identified from digital elevation models (via SRTM) and knowledge of dam operations;
- Identifying vegetation changes (via Figure 3C) and attributing them to natural or human causes – be these direct (land-use changes) or indirect (eg via climate change); and
- The global importance of the Sudd to climate change – a recent analysis found the wetlands to be an unexpectedly large source of methane, likely from microbial activity.
Once the environment factors are understood, we then proceed to untangle how they have or have not played a role in the conflict. This can be done by integrating a conflict dataset into the analyses, such as text mining the Armed Conflict Location & Event Data Project (ACLED) for events associated with the environment, as shown in Figure 3B and 3E. Further insight can be gained from ancillary EO datasets – for example VIIRS night-time light data (see Figure 3E) can be linked to population density or fuel availability.
Try it yourself
Although many technical barriers still exist to processing and analysing EO data, one positive change in recent years has been the proliferation of interactive online platforms that are easily accessible and understandable for the layperson.
A number of portals illustrate data on a phenomenon with a complex relationship to conflicts: deforestation. Syria has lost a quarter of its forested area since 2010 and, as the Global Forest Change visualisation in Figure 4A shows, in particular during the last four years. These losses are coupled to the conflict: collapse in governance and declining fuel access have allowed belligerents to open new and unregulated markets in fuel wood, charcoal and commercial logging. Meanwhile in Iraq, fighting and border-management burns also contribute to deforestation. The Middle East is at the forefront of climate change and cannot afford to lose already minimal tree cover, given the significant consequences for biodiversity, farming, floods, soil erosion, dust storms and desertification.
The conflict–deforestation relationship is less clear in Colombia (see Figure 4B). Although the conflict did exert an overall negative impact, the area of forest disturbance accelerated by 50 per cent in 2017 and 2018, following the peace agreement. ‘Gunpoint conservation’ by the Farc rebel group had ended, thus allowing drug cartels, large landowners and dissidents to exploit weak policy and start large-scale coca and cattle ranching farms, including in protected areas.
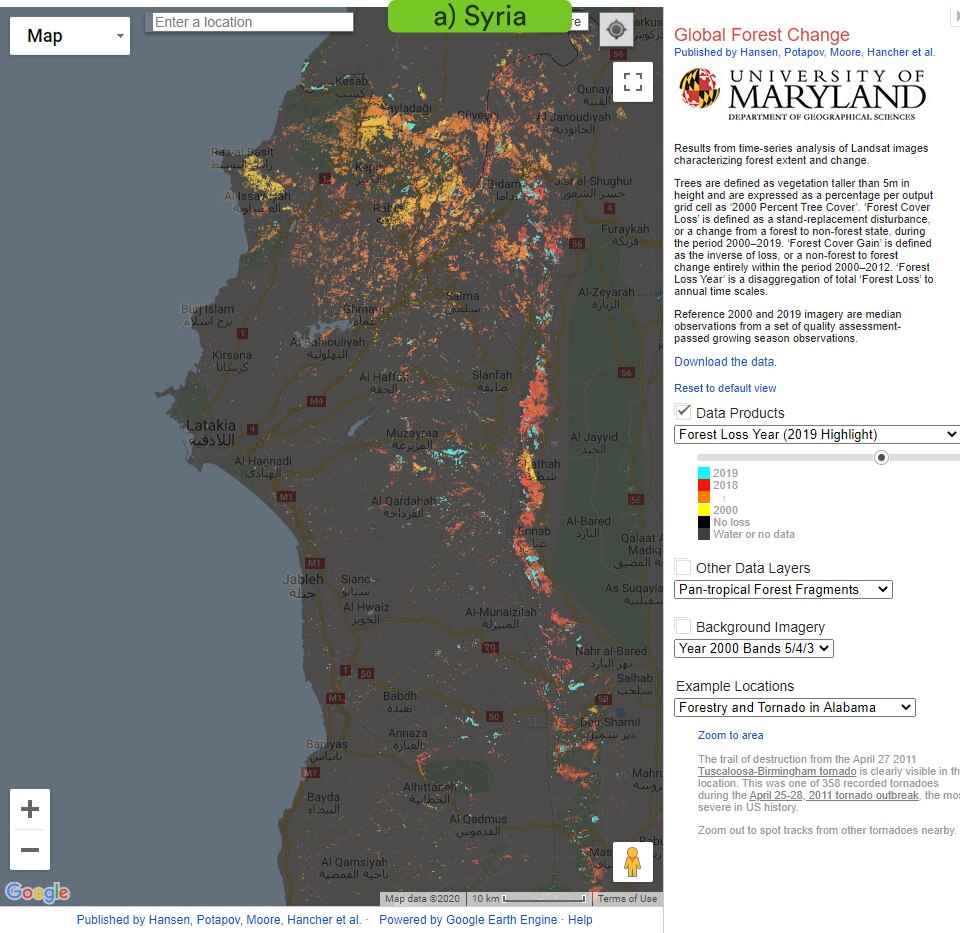
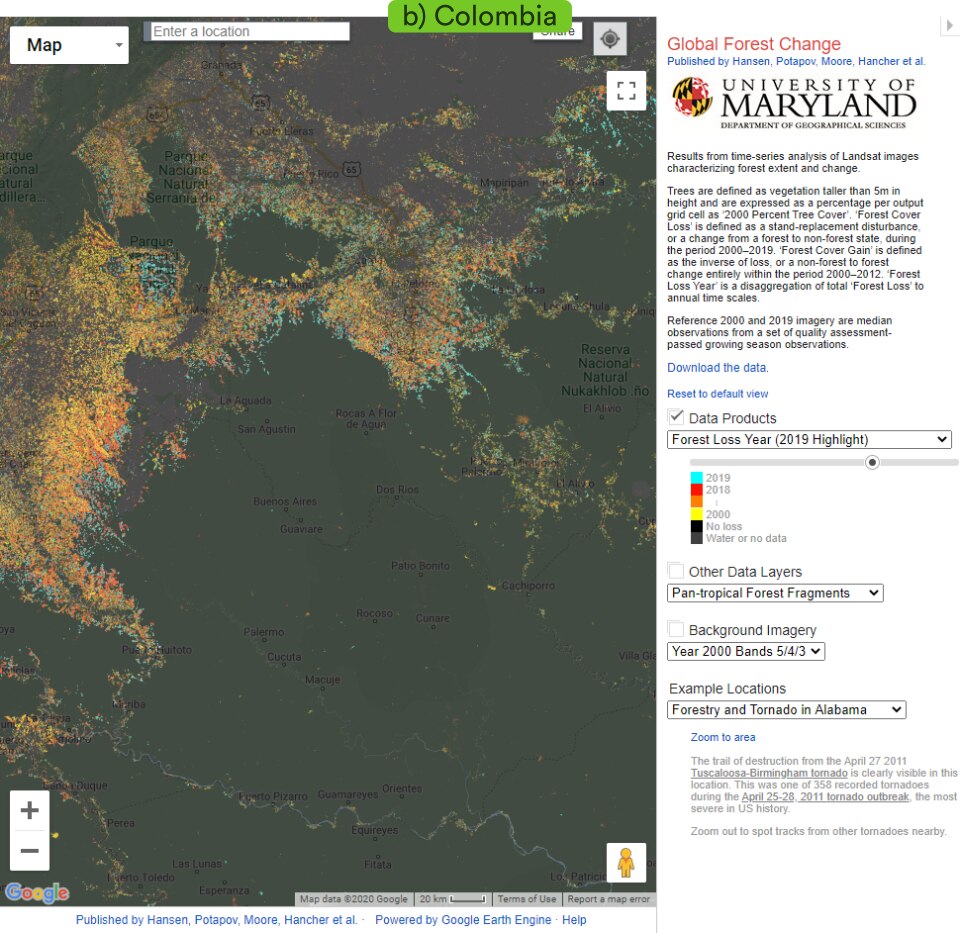
The apps.sentinel-hub.com EO browser is a powerful portal that allows users to choose from a host of sensor imagery collections, specify a region of interest, collate imagery from selected cloud-free dates and choose how it is presented. Most usefully, users can easily select band combinations to generate false-colour imagery, or write short snippets of code to manipulate the data or imagery.
Data come online approximately two days after the overpass, making the platform useful for near-real-time incident monitoring. The EO browser was helpful in quickly identifying and monitoring oil spilling into the Red Sea from the Sabiti tanker on 22 October 2019, following an attack on the vessel by unknown belligerents 11 days previously. Sentinel-1 SAR was processed using code available in the custom scripts repository to display water surface roughness (see Figure 5). The spill was significant in extent, yet there was no cleanup operation. The environmental damage is unknown, but the spill was close to endangered corals.
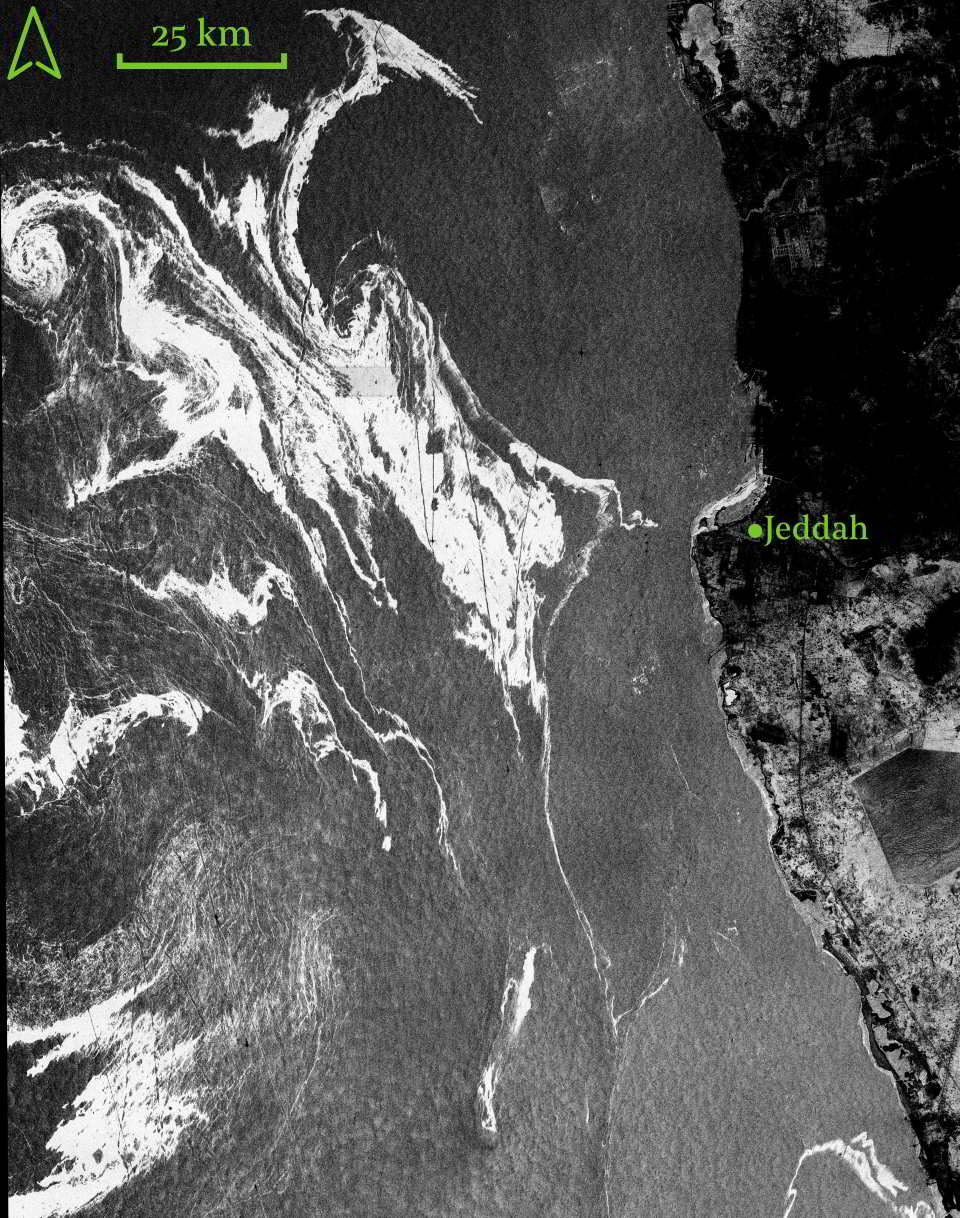
Ongoing challenges and future directions
As there is no commercial incentive and a misconception of unimportance, the field of observing the environmental dimensions of conflict with EO is small – it consists of a handful of non-governmental organisations (NGOs) and academics. Despite recent growth, which has helped to influence important progress in the legal, peacebuilding and policy spheres, there is still a need for sustained funding for environmental monitoring in regions at risk or affected by armed conflicts.
Assuming continued funding, the discipline ought to grow in step with the space sector, ensuring the application of frontier technology from new missions including BIOMASS, FLEX, NISAR, Landsat-9 and GeoCARB. Plans are in place to build the discipline, one route may be to the Group on Earth Observations framework to form a new working group and entrain experts from other relevant fields along with practitioners from conflict-affected countries. One objective would be to work with relevant legal actors to understand, and later implement, the minimum data standard and presentation style for admissible evidence in a court of law.
Given the huge scope of work, especially in the face of today’s diverse, intractable and dynamic conflicts, there needs to be a move towards automation and cloud computing. For incident monitoring, this could involve an algorithm that (1) trawls social media looking for events, (2) pulls out the relevant data and then (3) generates plots and statistics. Continuing assembly of a spectral and SAR fingerprint library will help identify surface pollutants and features with greater speed and accuracy. For long-term monitoring, automation will be through increased use of machine learning and artificial intelligence – these can also be trained to find emerging trends. Dealing with such vast volumes of data has logistical and ethical considerations and is all part of a wider discussion on building a digital ecosystem for the planet.
Dr Eoghan Darbyshire is CEOBS’ Researcher. A version of this article first appeared in the June 2020 edition of The Environmental Scientist, the journal of the Institute of Environmental Sciences.
Acronyms
AEOLUS: Atmospheric Dynamics Mission Aeolus;
CATS: Cloud-Aerosol Transport System;
FLEX: FLuorescence EXplorer;
GEDI: Global Ecosystem Dynamics Investigation;
GeoCARB: Geostationary Carbon Cycle Observatory;
GOSAT: Greenhouse Gases Observing Satellite;
GPM: Global Precipitation Measurement;
HYSPLIT: Hybrid Single Particle Lagrangian Integrated Trajectory;
JASON: Joint Altimetry Satellite Oceanography Network;
MODIS: Moderate Resolution Imaging Spectroradiometer;
NISAR: NASA-Indian Space Research Organisation Synthetic Aperture Radar;
PALSAR: Phased Array type L-band Synthetic Aperture Radar;
SAR: synthetic-aperture radar;
SEVIRI: Spinning Enhanced Visible and Infrared Imager;
SRTM: Shuttle Radar Topography Mission;
TanDEM-X: TerraSAR-X Add-On for Digital Elevation Measurement;
TROPOMI: TROPOspheric Monitoring Instrument;
VIIRS: Visible Infrared Imaging Radiometer Suite